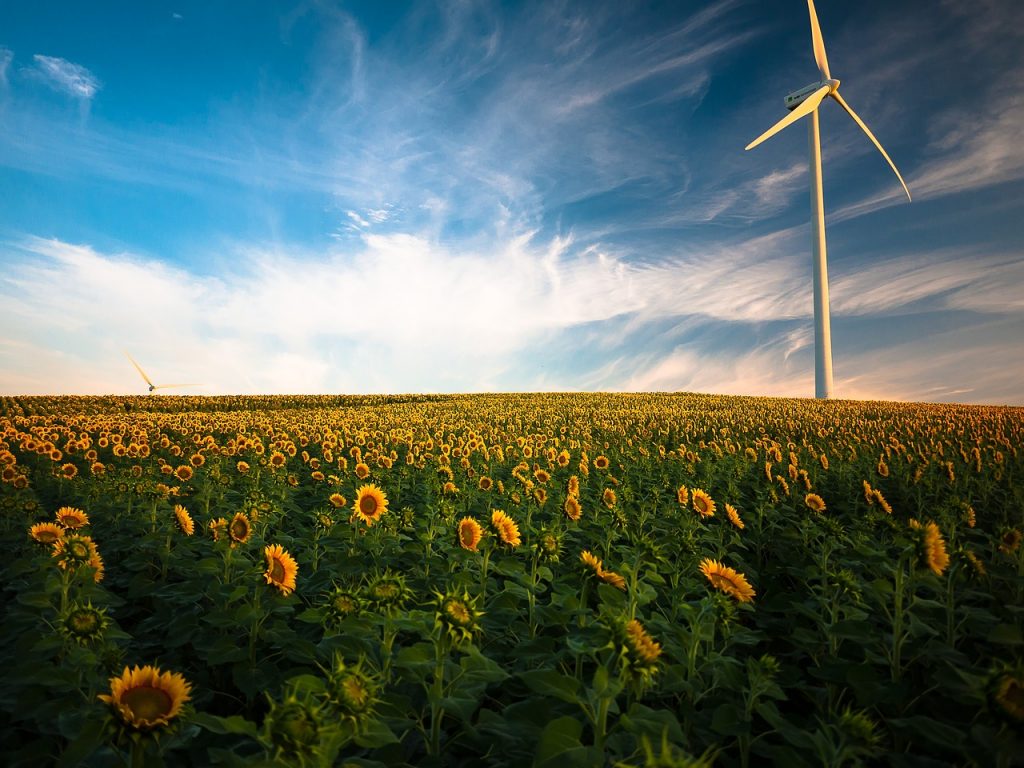
The long-term vision of the European Union (EU) to achieve climate neutrality by 2050 clearly entails a massive increase of the installed capacities of renewable energy sources and a simultaneous phase-out of fossil fuel consumption – both in the electricity and heat sector.
However, in particular the renewable electricity generation by mainly photovoltaics (PV) and wind energy is mostly fluctuating. In order to balance electricity generation and demand as well as avoid curtailment of PV plants and wind farms, a considerable increase of electrical energy storage (EES) capacities is required, which could then sum up to some 600 GWel in 2050.
Pumped hydro storage (PHS) is currently the most widely used EES technology in the EU and worldwide, but it has a limited potential of only about 150 GWel in Europe. Like PHS, compressed air energy storage (CAES) shows limited potential for further capacities due to the limited availability of suitable sites for the installation of such storage plants. In contrast to PHS and CAES, CHEST technology does not show these geographical constraints, and therefore has the potential to provide a relevant share of the massive additional EES capacities required, even though there are other EES technologies in place competing with CHEST. Besides the already mentioned PHS and CAES technologies, batteries will be a technology competing with CHEST, at least for some applications. Compared to batteries, the CHEST technology shows longer lifetimes, is less dependent on potentially scarce materials like lithium, and is expected to be less costly in very large-scale storage.
The switch from fossil fuels to RES in the heat sector will require additional storage capacities as well as a more efficient use of heat from various sources such as solar thermal, geothermal, biomass and waste heat. CHEST is an energy storage technology that takes up and stores both electricity and heat and thus can help increase the share of renewables in both the electricity and heat sector. Since it is a very flexible storage solution in terms of varying the electricity and heat output of the storage system, energy generation and demand can be balanced, and the electricity and heat sector coupled in a smart way.
In the CHESTER research project, the partners involved increased the maturity level of the CHEST technology by successfully developing, building and testing a first-of-its-kind prototype as well as conducting comprehensive theoretical analysis of this innovative technology.
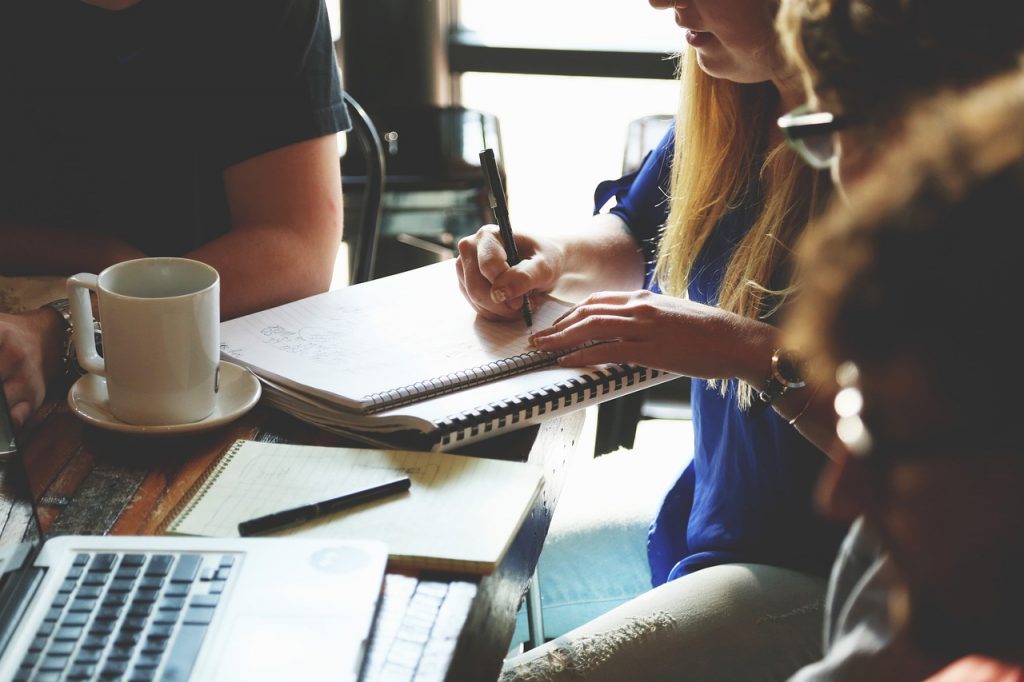
From a technological point of view, the next essential step towards the commercialisation of such CHEST systems is the scale-up from a charging and discharging power of currently 10 kWel up to several 100 kWel and finally to the MWel range. This can be realised by carrying out demonstration projects, where CHEST can also be tested in a real environment.
The project partners also identified key technological progress measures to be realised in further development. This applies to all three main components of a CHEST system: the high-temperature heat pump for the power-to-heat part, the high-temperature thermal energy storage system for the energy storage part and the organic Rankine cycle for the heat-to-power part. For instance, the combination of the HTHP and the ORC in one component or at least the common use of main parts of these two main components shows a high potential for a considerable reduction of investment costs, which was found to be inevitable for a future economic viability of CHEST. Furthermore, the latent heat storage, which is a part of the HT-TESS, was a first-of-its-kind development of a dual-tube concept. Further optimisation of this concept would reduce costs, as would the use of an alternative phase-change material (PCM). This would be possible with higher operating temperatures or through development and research of alternative materials.
From a non-technological point of view, it became clear that current boundary conditions for CHEST, but also for other EES technologies, are quite difficult in terms of achieving economic feasibility. This applies for instance to relatively low fluctuations of electricity prices or in general relatively low remunerations for the different electricity services an electrical energy storage can provide. When taxes or other financial burdens further reduce revenues realised by the provision of these electricity services, the revenues are often too low compared to the high investment costs of such storage systems.
For CHEST, these findings result in two major conclusions. First of all, when it comes to the provision of electricity services, only one service will very probably not be economically viable. Hence, CHEST should provide several of these services, such as energy arbitrage, the provision of response power or the use of CHEST in congestion management. Second, CHEST will be particularly interesting from an economic point of view if not only the charged and discharged electricity is utilised, but also the heat. If, for instance, industrial or other end-users require low-temperature heat, CHEST can provide this through heat generation by the ORC or directly from the thermal energy storage at a higher temperature level.
CHEST is an energy storage technology that couples the electricity and heat sector and has the potential to provide the storage capacities that will be so massively required in the coming decades mainly in the electricity, but also in the heat sector. Therefore, CHEST is expected to be a key energy storage technology in a future secure and decarbonised European energy system.
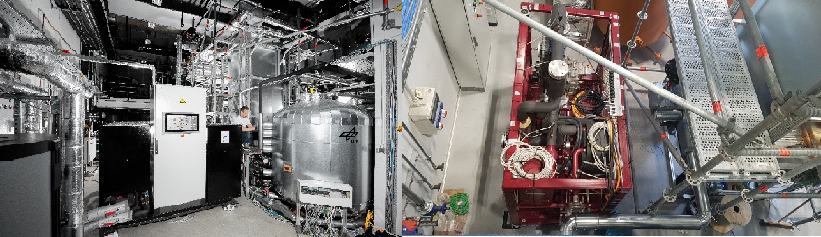